Bio-electromagnetics for Healthcare and Security Applications
Bio-electromagnetics examines the interaction between electromagnetic (EM) fields and biological organisms and tissues. Bioelectromagnetic studies within this group focus on state-of-the-art and novel methods to assess the interactions of EM fields across the spectrum with biological tissues, and develops means to develop new diagnostic and treatment technologies to serve today’s and future society. The group also focuses on EM compliance and safety assessments as well as improving the current standards by shedding further light on the assessment of EM properties of biological tissues and engaging with national and international committees and standard bodies to improve the current public guidelines.
The group’s interests include (not exclusive to):
-
EMF safety exposure assessments
-
Complex modelling of EM exposure and thermal patterns inside living systems
-
Assessments of dielectric properties of tissues across the frequency spectrum
-
Development of novel and state-of-the-art diagnostic and treatment technologies.
Our research aims to lead in innovation and in the development of more biologically-friendly, ecologically-sustainable and application-participator technologies and solutions.
Electromagnetic Interaction with Microparticles for Drug Delivery Systems
Rostyslav Dubrovka, Robert Donnan
The need for a sustained and better controlled drug administration has given rise to the development of novel drug delivery systems. A controlled drug release mechanism can help in maintaining drug dosage levels as well as optimise therapeutic effects and reduce side effects. Microparticles and nano-particles, initially developed as the carriers for vaccine and anticancer drugs, are now being used in drug delivery systems for drug targeting, improved release profile and increased efficiency. Therefore, this research involves the development of a remotely activated drug delivery system wherein electromagnetic interactions with metallic micro and nano-particles triggers the drug delivery

Schematic of Controlled Drug Delivery Implant consisting of split ring resonators as receivers of the incident microwave signals. The thermosensitive hydrogel is located near the gap regions of the resonators and metallic microparticles are embedded inside the hydrogel
The interaction of EM waves with small particles has enabled the development of advanced technologies like microscopic imaging, optical trapping, thermotherapy, micro and nanoparticle mediated drug delivery and cell penetration to name a few. However, the interaction of EM waves with matter at microwave frequencies is understood mostly for dielectric heating mechanism which occurs due to the electric field component of the EM radiation at a high frequency. But the microwaves also have a magnetic field component which also couples with certain metals in sufficiently small size and can generate heat. Thus, heating effects of EM radiation at the microwave frequencies can be due to dielectric heating, Joule heating, magnetic loss heating, etc. and the total power loss due to the heating effect of these EM waves can be obtained as a function of both the components of the electromagnetic field.
Therefore, metallic particles can be used as the agents for increasing temperature inside tiny blocks of materials such as smart polymers or thermosensitive hydrogels. The temperature responsive polymers respond quickly when there is an increase in temperature by a few degrees. Current simulations and theoretical studies in the research reveal that there is a considerable rise in temperature inside the polymers due to the interaction of the micro-particles with the electromagnetic radiation.

Incident microwaves heat the metallic particles causing a rise in temperature inside the hydrogel leading to contraction and release of the drug loaded particles.
Consequently, microwaves can be used for heating of metallic micro-particles embedded inside such smart hydrogels. The heating effect can then be utilised for externally triggered drug release. Further, the ISM band can be used in this technique for taking advantage of the already established standards regarding safety considerations and no license requirements. Thus, efficient design considerations can be used to design an effective drug delivery system which delivers low powered, non-ionising microwave signals to drug loaded implants inside the human body for controlled drug delivery. This work lays the foundation of the device to be used in many attractive applications such as drug delivery and remote triggering of electromagnetic microsystems.

S11 parameters and Simulated Electric field of the Modified SRR [1]
[1] Shaikh, M.S., Donnan, R.S., Dubrovka, R.F., "Heating Effect of the Electromagnetic Interaction with Metallic Microparticles", European Conf. on Antennas and Propagation, EuCAP 2021, paper #1570686908
Interaction with the Body across the frequency bands (SAR, SA and power density compliance Assessments)
Yasir Alfadhl, Xiaodong Chen
The Specific Absorption Rate (SAR) is used in dosimetry to denote the transfer of energy from the EM fields to biological materials (rate of energy deposition per unit mass of tissue).
While SAR is the measure of the incremental EM power absorbed by a given mass for continuous waves; the Specific Absorption (SA) [J/kg) is the quotient of the incremental energy absorbed by the incremental mass contained in a volume over a pulse duration.
With the increased interest in applications associated with higher frequencies above 6GHz, much more attention has been drawn to the assessments of the incident power densities to assess the incremental EM power density as it is absorbed by the surface of the body.

To demonstrate the need to shift focus to surface tissues when exposure is at higher frequencies, our studies have shown that E-field absorption are limited to skin layers, hence, the need to use power density assessments rather than the usual SAR assessments.
Our studies involve the utilisation of experimental and numerical methods to investigate the effects of EM wave absorption within tissues due to exposure to waves in the RF frequency band and beyond, and to pulsed waves. The SAR/SA induced within the human body, especially the head and brain, are typically evaluated by computing the absorption strength and the location of peaks/maxima.
Understanding the underlying interaction mechanisms caused by EM exposure is necessary for assessing the possible impact on human health. The interaction of EM fields with biological systems can be categorised by several mechanisms, depending on the type and frequency of exposure.

(a) (b) (c)
Examples of volume rendered images of the female model; (a) The outside surface; (b) Skin and skeleton; (c) Skeleton with a few internal organs (Skin, Fat, and Muscle removed); (d) 240 million FDTD/FIT voxels to compute the body accurately.
EM Absorption within the Body vs. Age
Yasir Alfadhl, Xiaodong Chen
Our studies include investigations on the effects of ageing on the level of interaction with EM fields, and whether such changes may have different implications on the human or animal well-being. Ageing variations have included the impact of body size changes, changes to dielectric properties due to ageing and other factors.
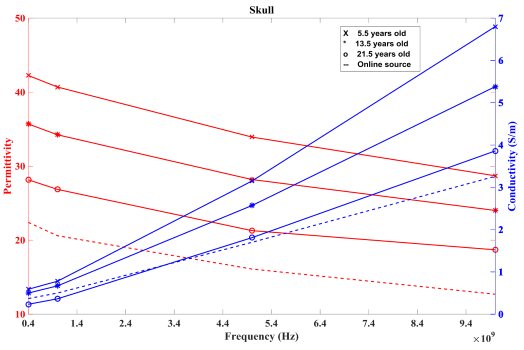
Example of comparison between age-dependent properties of skull tissue dielectric properties.
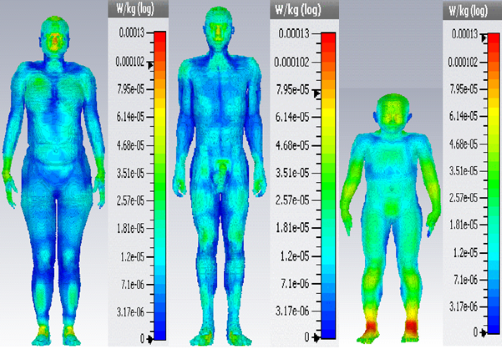
Examples body SAR(10) distributions due to planewave exposure at 868MHz, under sleeping condition. The figure represents the difference in peak values for female, male and child models.

SAR distribution resulting from exposure close to smart-meter antennas [1]; (a) whole-body SAR (WBSAR); (b) Max. SAR (10g); when models are under sleeping condition and exposed from top to bottom at 15cm distance.
[1]Qureshi, M. R. A., Alfadhl, Y., Chen, X., Peyman, A., Maslanyj, M., & Mann, S. (2018). "Assessment of exposure to radio frequency electromagnetic fields from smart utility meters in GB; part II) numerical assessment of induced SAR within the human body", Bioelectromagnetics, 39(3), 200-216. doi:10.1002/bem.22094
Macroscopic and Microscopic Electroporation Studies
Yasir Alfadhl, Xiaodong Chen
As part of the Electromagnetics and Antenna activities, the group has focused on the interactions of electromagnetic waves with biological matter (specifically on the cellular, sub-cellular and molecular levels), both experimentally and numerically. Electroporation is a non-thermal scheme based on applying short but intense pulses to the biological cells or tissues. The conductivity and permeability of the cell membrane can be increased dramatically during the process, which allows the large-sized molecules to flow through in both directions.
Molecular dynamics presents a microscopic view of electroporation that conventional observations cannot. Although the method has some challenges, it can be used to reveal some fundamental behaviour at the atomic level that is later confirmed from the experiment. Therefore, we have a number of studies where we combine the molecular dynamics observations with the experimental results to deepen the understanding of the electroporation process.
In our studies, we use evidence-supported-theory to describe the process of cellular electroporation by the means of experimental applicators with state-of-the-art sources and measurement methods, complemented with numerical computations of the molecular interactions with the fields applied (using Molecular Dynamics computation tools).
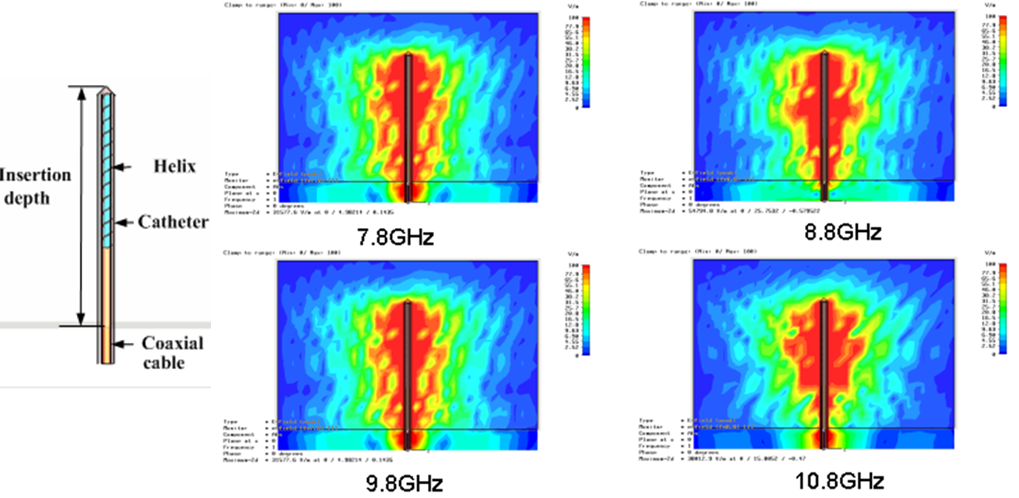
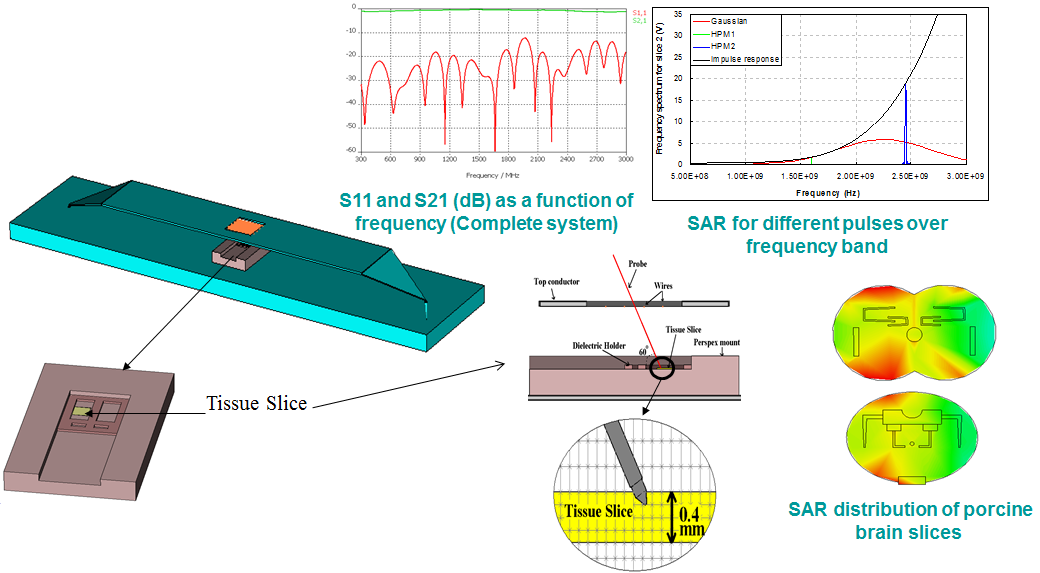
Assessment of dielectric properties and body composition
Yasir Alfadhl, Xiaodong Chen, Hasan Sagor
The interaction of EM waves with matter is depend on a number of parameters, one of which is the dielectric properties of the exposed material. In our studies, we apply various techniques to (i) study the effects of the variation of these properties within the exposed body; and/or (ii) estimate the characteristics of the exposed material by applying the inverse process. The latter is rather important, not only to predict the composition of the body (e.g., fat layers, etc.), but also to detect potential abnormalities within the body, such as cancerous regions. The techniques applied many include any of the impedance measurements, EM imaging, or dedicated scanning or radar techniques.
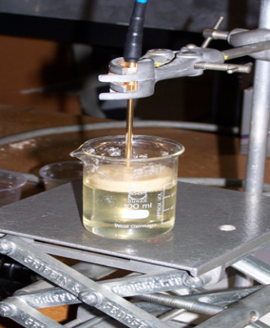
Calibration of the Agilent Dielectric Probe using deionised water.
Nano-particle Applications in Medical Fields
Research into the applications of Nano-particles (NPs) has found increased interest in diagnostics, imaging, and therapeutics in biology and medicine. In particular, Gold nano-particles (GNPs) have received much attention, because of their distinctive optical, electronic, and molecular-recognition properties. In medical applications, we look into the potential applications of GNPs for better targeting of EM-generated hyperthermia treatments of cancer. In essence, the EM illumination of GNPs can result in energy absorption and selectively heat and abolish tumour regions without affecting healthy tissue. In other studies, we explore the potential of targeted NPs for non-invasive ablation and drug delivery in radiofrequency (RF) EM fields. These studies aim to reveal the mechanism of GNPs heating under exposure, to study the molecule-targeting procedure of NPs, and to optimise the delivery of GNPs directed via novel antenna systems.

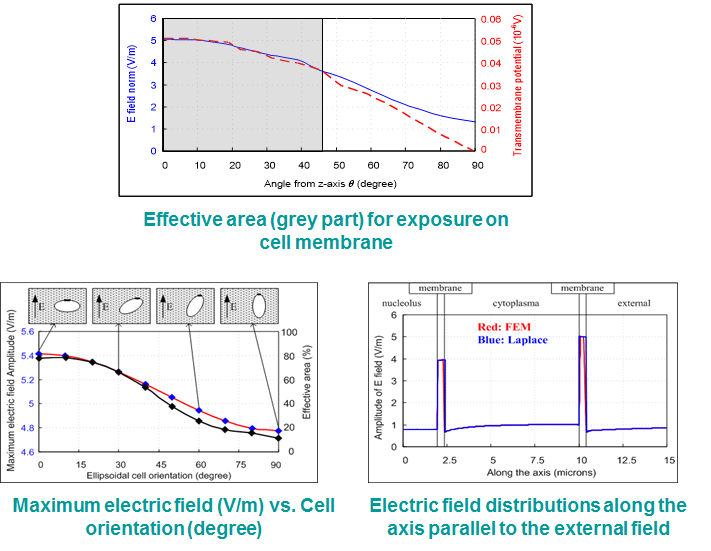

Other examples of Bio-EM projects
One example is the non-invasive monitoring of blood glucose levels (BGL). The electromagnetic properties of the body vary with BGL, which means the response of some resonator or EM device placed on the body will also vary, in theory, with the changes in BGL. Understanding this relationship is key to producing an EM sensor that can determine such physiological signals, which avoids the need for even minimally-invasive bio-sensor approaches. On the other hand, the use of ingestible or implantable wireless devices also requires an understanding of the interrelationship between the device and the surround tissue(s). Work in this area includes the development of digital and physical tissue phantoms (such as that in the header image above), the development and characterisation of microwave devices for wireless wearable physiological monitoring systems, and investigations to quantify the relationship between the various physiological parameters and the microwave response, including subject-specific variations.
The effect of biological tissues on electromagnetic waves can also be utilised for imaging purposes. The most well-known examples of this are X-ray imaging systems and the related X-ray Computed Tomography (CT) imaging systems. X-ray imaging finds application in healthcare (ranging from dentistry to imaging of the whole body), security (e.g., airport scanners) and industry (e.g., checking for structural integrity of pipes). However, X-rays are an ionising form of electromagnetic radiation, with associated health risks. Lower-energy non-ionising waves, such as at the THz, millimetre-wave and microwave frequency bands, can also be used for imaging, without the risks of X-rays.
Our studies in this domain include assessments of the effects of EM waves on the general public, EM biological anomalies detection methods, medical imaging schemes using EM waves, studies on the latest state-of-the-art medical treatments, and enhanced EM-assisted drug-delivery methods.
Highlights and Research Outcomes
-
The group’s work on the numerical dosimetry of the interaction of waves emitted from smart meters on the population (based on male, female and children numerical models) have been used by Public Health England to provide exposure safety guidelines for the public.
-
A range of novel devices and techniques have been developed to enhance the efficiency, accuracy, and to create state-of-the-art solutions to everyday challenges. Examples of such developments include (not exclusive to) novel exposure cavities, solid-state nano-second pulse generators for cancer treatments, novel near- and on- body communications and matched novel antennas for diagnostics and treatment.
-
The group’s contributions on the assessment of electromagnetic properties of biological tissues has resulted in increased accuracy of bioelectromagnetic problems and in assessing the interactions between electromagnetic fields and the human body.
-
The group's research activities in modelling and characterisation of Gold Nano-particles for medical treatment and diagnosis (Liu X, Chen HJ, Chen X et al., "Low frequency heating of gold nanoparticle dispersions for non-invasive thermal therapies", Nanoscale vol. 4 (13) 3945-3953, 2012) was cited and mentioned in a recent Science Review paper: Hong Koo Kim et al., "Are Gold Clusters in RF Fields Hot or Not?", Science 340, 441 (2013), DOI: 10.1126/science.1237303
Selected Research Grants and Projects
-
"Smart Meter Project", Public Health England (PHE) project on SAR assessments duet to Smart Meters (ITT_873) 2014/15
-
"Novel clinical nano-second electroporation device for cancer treatment", EPSRC IoB programme grant 2015.
-
Assessment of the interaction of EM signals from Smart-Meters on the general population” (Public Health England) 2014.
-
“Wearable and implantable antennas for in- and on-body applications”, 3Y-FT PhD studentship, 2014.
-
“Abdominal Fat Detection technique using EM waves”, 5Y-PT PhD studentship, 2014.
Selected Recent Publications
-
Sarjoghian, S., Rahimian, A., Alfadhl, Y., Saunders, T. G., Liu, J., & Parini, C. G. (2020). Hybrid development of a compact antenna based on a novel skin-matched ceramic composite for body fat measurement. Electronics (Switzerland), 9(12), 1-13. doi:10.3390/electronics9122139
-
Rao, X., Chen, X. D., Zhou, J., Liu, Y. Y., & Alfadhl, Y. (2020). Study on the Apoptosis Mechanism of Murine Melanoma B16 Cells Stimulated by Nanosecond Pulse Electric Field. Dianzi Keji Daxue Xuebao/Journal of the University of Electronic Science and Technology of China, 49(6), 949-954. doi:10.12178/1001-0548.2020099
-
Rao, X., Chen, X., Zhou, J., Zhang, B., & Alfadhl, Y. (2020). Design of a high voltage pulse generator with large width adjusting range for tumor treatment. Electronics (Switzerland), 9(6), 1-11. doi:10.3390/ELECTRONICS9061053
-
Sarjoghian, S., Alfadhl, Y., Chen, X., & Parini, C. G. (2021). A 3-D-Printed High-Dielectric Materials-Filled Pyramidal Double-Ridged Horn Antenna for Abdominal Fat Measurement System. IEEE Transactions on Antennas and Propagation, 69(1), 64-73. doi:10.1109/TAP.2020.3008653
-
Sarjoghian, S., Sagor, M. H., Alfadhl, Y., & Chen, X. (2019). A 3D-Printed High-Dielectric Filled Elliptical Double-Ridged Horn Antenna for Biomedical Monitoring Applications. IEEE Access, 7, 94977-94985. doi:10.1109/access.2019.2928629
-
Kothandaraman, A., Alfadhl, Y., Qureshi, M., Edirisinghe, M., & Ventikos, Y. (2019). Effect of the Mixing Region Geometry and Collector Distance on Microbubble Formation in a Microfluidic Device Coupled with ac-dc Electric Fields. Langmuir, 35(31), 10052-10060. doi:10.1021/acs.langmuir.8b03677
-
Qureshi, M. R. A., Alfadhl, Y., Chen, X., Peyman, A., Maslanyj, M., & Mann, S. (2018). Assessment of exposure to radio frequency electromagnetic fields from smart utility meters in GB; part II) numerical assessment of induced SAR within the human body. Bioelectromagnetics, 39(3), 200-216. doi:10.1002/bem.22094
-
Liu, X., Chen, H. J., Chen, X., Alfadhl, Y., Yu, J., & Wen, D. (2015). Radiofrequency heating of nanomaterials for cancer treatment: Progress, controversies, and future development. Applied Physics Reviews, 2(1). doi:10.1063/1.4915002
-
Liu, X., Chen, H. J., Chen, X., Alfadhl, Y., Yu, J., & Wen, D. (2014). Electromagnetic heating effect of aggregated gold nanoparticle colloids. Journal of Applied Physics, 115(9). doi:10.1063/1.4867615
-
Liu, X., Chen, H. J., Alfadhl, Y., Chen, X., Parini, C., & Wen, D. (2013). Conductivity and frequency dependent specific absorption rate. Journal of Applied Physics, 113(7). doi:10.1063/1.4791928
- Yilmaz T, Foster R and Hao Y (2014), "Broad-Band Tissue Mimicking Phantoms and a Patch Resonator for Evaluating Non-Invasive Monitoring of Blood Glucose Levels", IEEE Transactions on Antennas and Propagation, vol. xx, no. x, pp. xx-xx, DOI: 10.1109/TAP.2014.2313139