Metamaterials and Transformation Optics
Our metamaterials research started in 2001, focused on the microwave regime and with a strong emphasis on industrial applications. Our work has covered a very broad range of research topics in metamaterials, including electromagnetic bandgap structures, high impedance surfaces and partially reflective surfaces. Our work on left-handed metamaterials is based mainly on numerical modelling. Due to the dispersive nature of metamaterials, the conventional FDTD method requires modification to tackle both frequency and spatial dispersions. Once the limitations of left-handed metamaterials were uncovered (for instance, limited bandwidth and high losses), we investigated many alternative materials and structures to enable applications, such as directive and compact antennas, subwavelength imaging and broadband microwave systems. We also developed techniques to model invisibility cloaks. Meanwhile, it has offered us an opportunity to work on the exciting concept of transformation optics/electromagnetics.
From 2011-1026, we led the EPSRC Programme Grant “QUEST”, with the aim to apply both transformation optics and metamaterials to practical applications at microwave frequencies. This included theoretical work, fundamental work on materials fabrication and investigation of numerical design tools, as well as numerous applications. Following the completion of QUEST, we were involved in 2 further EPSRC funded projects. AOTOMAT developed adaptive tools for electromagnetic and materials modelling to bridge the gap between design and manufacturing. The SYMETA project investigated new methods for synthesizing metamaterials at microwave to THz frequencies. Our current EPSRC portfolio includes the ANIMATE project, which is focussed on the development of software defined materials for active EM technology.
Computational Electromagnetics and Optimisation including Machine Learning
Yang Hao, Henry Giddens
Computer modelling of electrodynamics, materials and electronics has always been an important field, as it enables the industry and also academic users from other disciplines to capture newly developed theory and transform them seamlessly into commercial products and engineering applications. Our group has a long tradition of studying different numerical techniques to solve the Maxwell's Equations. We started it with the development of FDTD algorithm in the nonorthogonal coordinate system. We also developed some novel techniques to tackle both frequency and spatial dispersion of metamaterials. We developed the first time domain simulation of invisibility cloak, where superluminal behaviour of wave propagation can be visualised. While commercial modelling tools are becoming more and more powerful, we have now turned our attention to the modelling of novel materials and devices. For example, we have developed FDTD algorithms to understand the nonlinearity and THz responses of graphene under DC magnetic fields. We have also stretched our knowledge to include the Monte Carlo technique to model electron transport in graphene and other 2D materials. This has helped us to develop accurate transistor models, which can be used in the design of novel active devices and metamaterials. More recently, we have implemented a coupled nonlinear model to study tunable metamaterials containing ferroelectrics and accurately predict their effective properties as a function of an external applied voltage, taking into account the localized electric field enhancement in the unit cell.
Materials by design has always been perceived as a scientific fiction and now with the rapid advance of machine-learning, and fast modelling tools, it has become viable to tailor-make materials ranging from nanocomposites, graphene and 2D materials, metamaterials. This allows us to look into the possibility of developing new devices and systems across different length scales. One of examples is that, based on the dipole moment technique, we are now able to model up to 30,000 nanoparticles inside a Luneburg lens. We have also developed topology optimisation algorithms to design metamaterials with complex geometries according to performance objectives and manufacturing constraints, and applied them to invisibility cloaks and illusion devices. This will no doubt provide us the opportunity to apply different optimisation techniques to modify our designs according to industrial requirements.
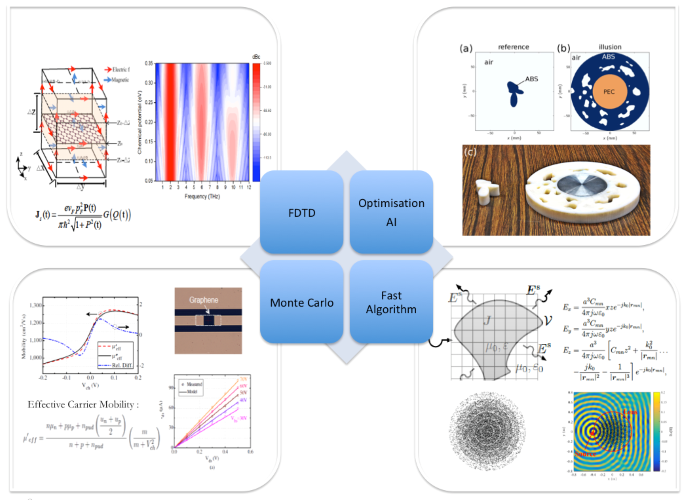
Figure: Closing the loop from design to manufacture using novel modelling techniques, optimisation and machine learning and additive manufacturing for realisation of high-performance EM devices
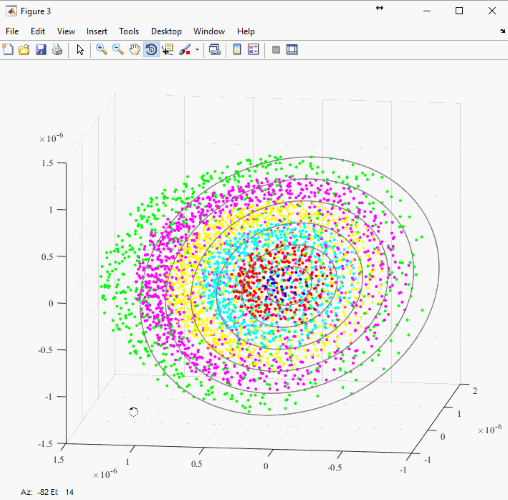
Figure: A Composite Luneburg lens modelled with 30,000 nano-particles using the dipole moments technique
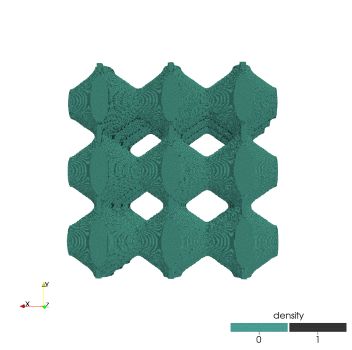
Figure: Optimised meta ferro-electric ceramics. The dark material has an electric-field dependent dielectric constant. By optimising the shape of the metamaterial at a sub-wavelength scale, we are able to increase the effective tunability of the material
Highlights and Research Outcomes
- The group has published a book, more than 4 book chapters and more than 100 substantial journal and refereed conference papers on Metamaterials and Transformation Optics.
- This work is in collaboration with many academic partners (University of Birmingham, University of Oxford, University of Exeter, University of St Andrews, etc.) and industrial collaborators (DSTL, USA Office of Naval Research, BAE Systems, ERA Technology, etc.).
- The group has organised or co-organised two conferences, 3 workshops and two short courses related to Metamaterials and Transformation Optics.
- Academics from the Group have been invited to deliver special talks and keynotes in many international conferences organized by the IEEE and IET
Selected Research Grants and Projects
-
2013.10 – 2016.9, Industrial CASE award on graphene and magnetic metamaterials, £130K, BAE Systems
-
2011.7 – 2016.6, The Quest for Ultimate Electromagnetics using Spatial Transformations (QUEST) in total £5M, EPSRC, with Universities of Oxford and Exeter
-
2016 - 2021, SYnthesizing 3D METAmaterials for RF, microwave and THz applications (SYMETA) – EPSRC
-
2016 - 2019, Adaptive Tools for Electromagnetics and Materials Modelling to Bridge the Gap between Design and Manufacturing (AOTOMAT) - EPSRC
-
2018 - 2022, SOFTWARE DEFINED MATERIALS FOR DYNAMIC CONTROL OF ELECTROMAGNETIC WAVES (ANIMATE) – EPSRC
-
2015 - 2018 and 2019 - 2022, Frequency Agile Antennas for Software Defined Radio (DALMATION) – DSTL
Selected Recent Publications
- Orestis Christogeorgos, Haoyang Zhang, Qiao Cheng, Yang Hao, “Extraordinary Directive Emission and Scanning from an Array of Radiation Sources with Hyperuniform Disorder”, Physical Review Applied 15 (1), 014062, 2021
- H Zhang, H Gidden, TG Saunders, Y. Hao et. al, “High Tunability and Low Loss in Layered Perovskite Dielectrics through Intrinsic Elimination of Oxygen Vacancies”, Chemistry of Materials 32 (23), 10120-10129, 2020
- H Zhang, H Giddens, Y. Hao et. al, “Polar nano-clusters in nominally paraelectric ceramics demonstrating high microwave tunability for wireless communication”, Journal of the European Ceramic Society 40 (12), 3996-4003, 2020
- Y Ma, Y Hao, “Antenna Classification Using Gaussian Mixture Models (GMM) and Machine Learning”, IEEE Open Journal of Antennas and Propagation 1, 320-328, 2020
- H Giddens, Y Hao, “Multibeam graded dielectric lens antenna from multimaterial 3-D printing”, IEEE Transactions on Antennas and Propagation 68 (9), 6832-6837, 2020
- B Liu, H Giddens, Y Li, Y He, SW Wong, Y Hao, “Design and experimental demonstration of Doppler cloak from spatiotemporally modulated metamaterials based on rotational Doppler effect”, Optics express 28 (3), 3745-375, 2020
- Ning Liu, Achintha Ihalage, Hangfeng Zhang, Henry Giddens, Haixue Yan, Yang Hao, “Interactive human–machine learning framework for modelling of ferroelectric–dielectric composites”, Journal of Materials Chemistry C 8 (30), 10352-10361, 2020
- B Vial, Y Hao, “Enhanced tunability in ferroelectric composites through local field enhancement and the effect of disorder”, Journal of Applied Physics 126 (4), 044102, 2019
- Baiyang Liu, Hongchen Chu, Henry Giddens, Ronglin Li, Yang Hao, “Experimental observation of linear and rotational doppler shifts from several designer surfaces”, Scientific reports 9 (1), 1-9, 2019
- Q Cheng, M Naeem, Y Hao, “Composite Luneburg lens based on dielectric or plasmonic scatterers”, Optics express 27 (8), 10946-10960, 2019
- H Shi, H Giddens, Y Hao, “Field transformation-based multifunctional and wide-angle polariser for antenna polarisation characteristics manipulation”, IET Microwaves, Antennas & Propagation 13 (9), 1450-1456, 2019
- L La Spada, C Spooner, S Haq, Y Hao, “Curvilinear metasurfaces for surface wave manipulation”, Scientific reports 9 (1), 1-10, 2019
- H Zhang, H Chu, H Giddens, W Wu, Y Hao, “Experimental demonstration of Luneburg lens based on hyperuniform disordered media”, Applied Physics Letters 114 (5), 053507, 2019
- PJ Bradley, MOM Torrico, C Brennan, Y Hao, “Printable all-dielectric water-based absorber”, Scientific reports 8 (1), 1-11, 2018
- J Lei, J Yang, X Chen, Z Zhang, G Fu, Y Hao, “Experimental demonstration of conformal phased array antenna via transformation optics”, Scientific reports 8 (1), 1-13, 2018
- L La Spada, S Haq, Y Hao ,”Modeling and design for electromagnetic surface wave devices”, Radio Science 52 (9), 1049-1057, 2017
- BY Wu, XQ Sheng, Y Hao, “Effective media properties of hyperuniform disordered composite materials”, PloS one 12 (10), e0185921, 2017
- H Shi, H Giddens, Y Hao, “Dual-circularly polarized patch antenna using field transformation medium”, IEEE Antennas and Wireless Propagation Letters 16, 2869-2872, 2017
- B Vial, MM Torrico, Y Hao, “Optimized microwave illusion device”, Scientific reports 7 (1), 1-8, 2017
- B Vial, Y Liu, SAR Horsley, TG Philbin, Y Hao, “A class of invisible inhomogeneous media and the control of electromagnetic waves”, Physical Review B 94 (24), 245119, 2016
- B Vial, Y Hao, “A coupling model for quasi-normal modes of photonic resonators”, Journal of Optics 18 (11), 115004, 2016
- L La Spada, TM McManus, A Dyke, S Haq, L Zhang, Q Cheng, Y Hao “Surface wave cloak from graded refractive index nanocomposites”, Scientific reports 6 (1), 1-8, 2016